I once read about a breeder
who tried to breed blue roan Texas Longhorns, but gave up after trying
for several years with little success. He stated that the inheritance
of color just wasn’t predictable in the breed. In truth, producing
blue roans is not difficult, but the trick is that the best way to achieve
this result is not by breeding two blue roans together. This month, I’ll
discuss this topic as we examine the genetics of a few more white-producing
genes, including Brockling and Roan, and I’ll
also discuss some of the unusual ways that the various white-producing
genes can interact with one another.
Technically, the Brockling gene is not a white-producing gene,
but its effects are only seen in combination with the various white-producing
genes. The dominant brockling allele (Bc) interacts with other
genes to produce pigmentation on areas of the body that would otherwise
be white (especially on the extremities, including the legs and the head).
The most common pattern observed in Texas Longhorns is in animals that
are homozygous s/s for the Spotting gene. Recall that
this genotype produces animals that are spotted (with spots varying from
large to small), and normally the legs and head would be unpigmented or
spotted. But in the presence of the Bc allele at the Brockling
gene, the animals appear to be wearing knee-socks (in other words, their
legs are mostly pigmented) and a hood (a pigmented head and neck; see
Figure 1). If the animal is homozygous for the normal recessive allele
at the Brockling gene (e.g., bc+/bc+), then the legs
and head will not be fully pigmented. The Bc allele can also
produce pigmentation on other parts of the body, and often results in
larger spots of coloration on the trunk.
Whereas alleles of the Brockling gene can increase the areas
of coloration, alleles of the Roan gene can reduce overall pigmentation.
The incompletely dominant R allele of the Roan gene
restricts pigmentation (both phaeomelanin, or red pigment, and eumelanin,
or black pigment). Texas Longhorns that are homozygous red (e/e
at the Extension gene; see Part 1 of this series) and heterozygous
R/r+ at the Roan gene will be red roans (a mixture of
red and white hairs will be produced; Figure 2). Likewise, cattle that
have at least one copy of the dominant black (ED)
allele at the Extension gene and are heterozygous R/r+
at the Roan gene will be blue roans (a mixture of black and white
hairs; Figure 3). Animals that have wild-type coloration (E+/e or
E+/E+ at the Extension gene) and are also heterozygous
R/r+ at the Roan gene are somewhat variable (typical
of wild-type coloration), but normally exhibit some type of “wine
colored” roan pattern (Figures 4 and 5). Wine roans are also called
purple roans or mulberry roans, and vary from dark purple (as in Figure
4) to a lighter mulberry (as in Figure 5). This variation is typical of
that produced by the wild-type allele at the Extension gene,
and is related to the relative production of the two pigments (red phaeomelanin
and black eumelanin; see Parts 1 and 3 of this series). The relative intensity
of the purple roan coloration in these animals probably is related to
interactions with other genes, although the details of these interactions
are not fully understood. Although heterozygosity for the R allele
at the Roan gene produces red, blue, and purple roans, homozygosity
for the R allele produces an almost completely white Texas Longhorn
(with pigmented ears), no matter which alleles are present at the Extension
gene.

Figure 1. A Texas Longhorn cow (Golden Counter) that shows the effects
of the dominant brockling allele (Bc). Notice the pigmented legs,
head, and large spots of color on the body.
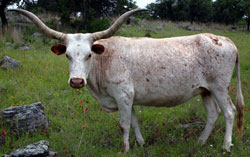
Figure 2. Red roan (Jackie Lynn 2916).
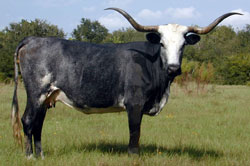
Figure 3. Blue roan
(Ms. Blue Butler, photo courtesy of Malcolm Goodman).
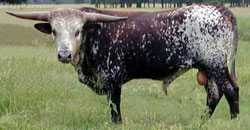
Figure 4. A wine roan (also called a purple roan or mulberry roan) Texas
Longhorn bull
(Dalgood’s Mojo Rocket, photo courtesy of Malcolm Goodman).
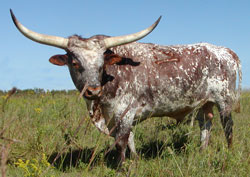
Figure 5. Another (lighter) wine roan Texas Longhorn bull.
(Roundup’s Purple Sage, photo courtesy of Gary and Lisa Baugher).
However, as I briefly mentioned last month, all roans
are not produced from the Roan gene. In addition, blue roans
can be produced when animals have at least one copy of the black allele
(ED) at the Extension gene and
are heterozygous at the Color-sided gene (Cs/cs+). Therefore,
there are two distinct genetic mechanisms for producing blue roans. The
color-sided allele is common among Butler cattle, but true black Butler
cattle are uncommon. Therefore, the combination of black with either a
heterozygous Color-sided or a heterozygous Roan animal
is relatively rare, at least compared to some other color patterns. Moreover,
if the animal has both the R allele at the Roan gene
and the Cs allele at the Color-sided gene, or both the
R allele at the Roan gene and the Sp
allele at the Spotted gene, or both the Cs allele at
Color-sided and the Sp allele
at Spotted, then it will be almost all white, except for pigmented
ears and perhaps a few small spots of color (the White Park pattern).
A mostly white animal is also produced if the animal is homozygous R/R
at the Roan gene, or homozygous Cs/Cs at the color-sided
gene. Therefore, there are a large number of genetic combinations that
produce white animals with pigmented ears, which is why some people mistakenly
think of white coloration as dominant.
Let’s now return to the problem of breeding for blue roans. It would
be natural to attempt to breed for blue roans by selecting a blue roan
bull and breeding it to roan cows (probably red roans, since initially
a breeder might not have access to many blue roan cows). Table 1 shows
the expectations of a cross of a blue roan bull (ED/e
at Extension, Cs/cs+ at Color-sided, and r+/r+
at Roan) to a red roan cow (e/e at Extension,
cs+/cs+ at Color-sided, and R/r+ at Roan).
Note that we would expect to get a wide variety of colors and patterns
of offspring, including blue roans, red roans, solid blacks, solid reds,
white with red ears, white with black ears, and color-sided red animals!
After a decade or so of breeding these two animals, we might expect to
get a couple of blue roan offspring, but even these blue roans could have
this coloration because of a different genetic basis from one another.
It would be natural to now select a blue roan bull and a blue roan cow
from this cross, and try again to breed for blue roan offspring. However,
if we selected one of each of the two genotypes that produce blue roans
from the first cross, then we would expect the results shown in Table
2. Notice that the expectations of this cross (now of two blue roans)
still include blue roans, red roans, solid blacks, solid reds, white with
black ears, white with red ears, and color-sided red animals. After all
this effort, the frequency of blue roans has gone from one-quarter of
the offspring up to only three-eighths of the offspring. At this point,
most breeders would probably give up, after many years of attempts to
breed for blue roans with only occasional success.
Table 1. The expectations of a cross of a
blue roan bull ( ED/ e
at Extension, Cs/cs+ at Color-sided,
and r+/r+ at Roan) to a red roan cow ( e/e
at Extension, cs+/cs+ at Color-sided,
and R/r+ at Roan).
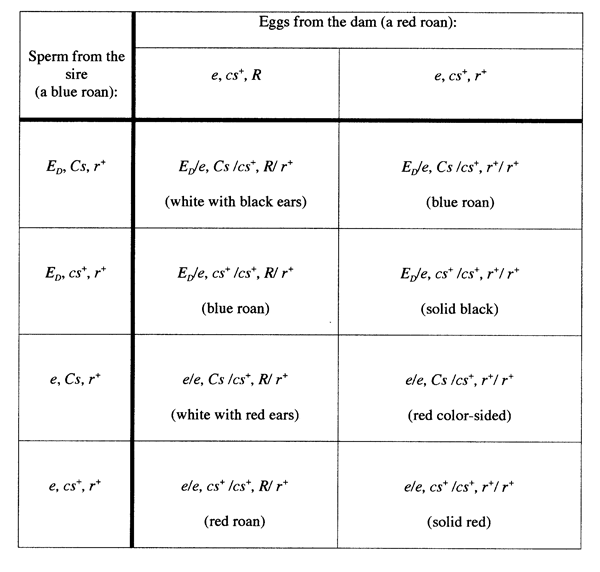 |
Table 2.The expectations of a cross of a blue
roan bull ( ED/ e at Extension,
Cs/cs+ at Color-sided, and r+/r+ at
Roan) to a blue roan cow ( ED/ e
at Extension, cs+/cs+ at Color-sided,
and R/r+ at Roan).
|
Despite this discouraging example, breeding for blue roans is actually
quite simple. However, the trick is that the best success does not come
from breeding blue roan bulls with blue roan cows. Instead, if we start
with a bull that is all white except for black ears, and is homozygous
ED/ED
for the Extension gene, homozygous cs+/cs+ for the Color-sided
gene, homozygous S+/S+ for the Spotted gene, and homozygous
R/R for the Roan gene, and mate this bull to any solid
black, red, or wild-type (e.g., brindle or Parker brown) cow, then ALL
of the offspring would be expected to be blue roans! This is because all
of the offspring would carry at least one black (ED)
allele at the Extension gene (recall that black is dominant over
the other colors), would be homozygous cs+/cs+ for the Color-sided
gene and homozygous S+/S+ for the Spotted gene, and
would be heterozygous R/r+ for the Roan gene, thus resulting
in blue roan coloration. One of the reasons that blue roans are not more
common is that many breeders are hesitant to select mostly white bulls
for use as herd sires, because the breeders think that white bulls will
not produce colored offspring. As this example shows, however, this belief
is not necessarily correct. The color of the bull is not as important
for herd sire selection as is its genetic basis for that color. Since
there are many different genetic reasons why a given bull might be white,
some knowledge of the ancestors of the bull and the colors that the ancestors
exhibited is needed to predict the expected colors of the bull’s
offspring.
As I mentioned last month, many breeders also avoid mostly
white cows, and white cattle tend to bring lower prices at auction. However,
as the example above demonstrates, some white cattle can produce a wide
variety of offspring, including solid, brindled, roaned, linebacked, color-sided,
and spotted calves. They can also produce white offspring, of course,
especially if the selection of mates is not made carefully. For instance,
the bull Classic
had the reputation of producing many white calves, although he also produced
many colored offspring (especially color-sided). This was because Classic
was homozygous for the color-sided allele (Cs/Cs), which results
in the White Park pattern. Since many breeders used Classic
in combination with other Butler cattle, and since the color-sided allele
is quite common in the Butler family, many of these offspring were also
homozygous Cs/Cs, and therefore white. However, breeding Classic
to solid colored (red, black, or wild-type) cows produces color-sided
offspring (heterozygous Cs/cs+).
In this five-part series, I’ve attempted to present our current
state of knowledge concerning the genetics of Texas Longhorn coloration.
There are undoubtedly other genes, in addition to the ones that I’ve
discussed here, that affect coloration, so I would welcome information
on color patterns that do not seem to fit the genetic explanations that
I’ve presented. In a future article, I plan to present and will
attempt to answer any questions that I receive about Texas Longhorn coloration,
and I will also present examples of additional types of coloration that
readers bring to my attention. So, if you have questions or examples that
do not seem to fit the genetic explanations I’ve presented in this
series, please send me an e-mail or letter.
I’ve presented information on eight genes that are known to influence
color variation in Texas Longhorns (Extension, Brindle, Dilution,
Dun, Spotted, Color-Sided, Brockling, and Roan), and each
of these genes has two or three different alleles that exist in Texas
Longhorn herds. As I mentioned in Part 1 of this series, there are 26,244
different possible genetic combinations of these alleles. This helps explain
why, as J. Frank Dobie so clearly noted in The Longhorns, that the colors
of Texas Longhorns are “more varied than the colors of the rainbow”.
I hope that a better understanding of the genetic origins of these varied
colors and patterns will aid breeders who wish to breed for particular
combinations.
Acknowledgements: I thank Gary and Lisa Baugher, Malcolm
Goodman, and Rex Mosier for allowing me to use photographs of their cattle
for this article.
Want more detail? Please see the following papers:
Additional
Reading and References
Hillis,
D. M. 2004. The genetics of coloration in Texas Longhorns: Part 1: The
basic colors. Texas Longhorn Trails 16(3):40-41.
Hillis,
D. M. 2004. The genetics of coloration in Texas Longhorns: Part 2: Grulla,
dun, and other reduced pigment patterns. Texas Longhorn Trails
16(5):76-77.
Hillis,
D. M. 2004. The genetics of coloration in Texas Longhorns: Part 3: The
wild-type color variants. Texas Longhorn Trails, September 2004.
Hillis,
D. M. 2004. The genetics of coloration in Texas Longhorns: Part 4: Spotted,
lineback, color-sided, and White Park patterns. Texas Longhorn Trails,
October 2004.
Joerg, H.
et al. 1996. Red coat color in Holstein cattle is associated with a deletion
in the MSHR gene. Mammalian Genome 7: 317-318.
Klungland,
H. et al. 1995. The role of melanocyte-stimulating hormone (MSH) receptor
in bovine coat color determination. Mammalian Genome 6: 636-639.
Lauvergne,
J. J. 1966. Génétique de la couleur de pelage dex boivins
domestiques. Bibliographia Genetica 20:1-168.
Olson, T.
A. 1980. Choice of a wild-type standard in color genetics of domestic
cattle. Journal of Heredity 71:442-444.
Olson, T.
A. 1981. The genetic basis for piebald patterns in cattle. Journal
of Heredity 72:113-116.
Olson, T.
A. 1999. Genetics of Colour Variation. In The Genetics of Cattle
(R. Fries and A. Ruvinsky, eds.). Pp. 33-53. CABI Publishing, Wallingford,
United Kingdom.
Robbins, L.
S. et al. 1993. Pigmentation phenotypes of variant extension locus alleles
result from point mutations that alter MSH receptor function. Cell
72:827-834.
|