The
diversity of Texas Longhorn coloration is celebrated by many modern longhorn
breeders. Texas Longhorns represent one of the best breeds in which to
study the genetics of coat coloration, because much of the color variation
that is known across all breeds of cattle exists in Texas Longhorns. However,
this great diversity of color leaves many people baffled. I have heard
some breeders say that they selected a bull because it exhibited a particularly
desirable color pattern, but then they discovered that none of the bull’s
offspring seemed to exhibit the same coloration. This leads some people
to state the common (but false) expression that “you can’t
breed for color in Texas Longhorns.” On the other hand, some breeders
will guarantee that their bull will produce calves with black coloration.
Clearly, then, it is possible to breed for color in Texas Longhorns, but
predicting color is not always simple. Why do some bulls (and cows) produce
calves of predictable colors, whereas others do not?
Basic Genetics
The physical appearance of a Texas Longhorn (or any other animal) is determined
by both genetic and environmental factors. The appearance of some features,
such as coloration, is determined almost exclusively by genetics. The
appearance of other features, such as horn length, is determined by a
combination of genetics and environment.
The genetic component of a Texas Longhorn’s appearance is determined
by its genome (the total of all its genes), which is in turn composed
of DNA (the “double helix”
molecule).
The genome of an organism is like an instruction book that specifies what
the animal will look like. In the language of the genome, all the words
are spelled with just four letters. Furthermore, in this simple language,
all the words are just three letters long. This is the language of the
double helix: the four building blocks
(the “letters” of the language) are called nucleotides, and
are typically represented by the letters A, C, G, and T. A gene is simply
a long string of these four nucleotides. Each combination of three nucleotides
(called a codon) specifies a particular amino acid (the building blocks
of proteins). Proteins, in turn, control almost everything about the way
an organism is constructed and the way it functions. Different proteins
are simply different combinations of amino acids.
A gene is a particular region of the genome that provides the instructions
for building a given protein. Small differences in these proteins produce
the genetic differences we observe among Texas Longhorns. These proteins
control how the animal is built, how it functions, and what it looks like.
Every Texas Longhorn inherits two copies (with a few exceptions) of each
of its genes—one copy from the dam, and one from the sire. Small
differences in these copies result in different forms of the genes, called
alleles. If a cow has two copies of the same allele, the cow is said to
be homozygous for that gene. If the two copies are different, the cow
is heterozygous for that gene.
There are eight genes that are known to influence color variation in Texas
Longhorns, and each of these genes has two or three different alleles
that exist in Texas Longhorn herds. There are 26,244 different possible
genetic combinations of these alleles that can occur in any given Texas
Longhorn cow or bull, which partly explains why there is so much variation
in color in this breed. However, we can break these combinations down
into a much smaller number of color classes, and consider these genes
one at a time. This allows us to make predictions about the possible colors
of calves that may result from a particular breeding.
Over the next several months, I’ll discuss each of these genes and
how it affects coloration in Texas Longhorns. This month, we’ll
start with a discussion of the three basic colors of Texas Longhorns that
are controlled by a single gene. In future months, I’ll discuss
how various patterns with white markings are produced, as well as topics
such as the genetics of colors such as brindle, grulla, dun, and roan.
If you are interested in a particular color pattern (grulla, for instance),
it is often possible to select cows and bulls that will produce only grulla
coloration, as long as you understand the underlying genetics.
The Basic Colors
Given the diversity of colors seen in Texas Longhorns, many people are
surprised that there are just two different pigments that produce all
of the hair colors in cattle (and for that matter, all mammals). These
two pigments are eumelanin (black) and phaeomelanin (red). Eumelanin is
a black pigment, but also looks brown or grey in lower concentrations.
Phaeomelanin is a red pigment, but can look orange or yellow in lower
concentrations. If neither pigment is produced, then hair is white. Therefore,
all of the roans, brindles, speckled patterns, linebacks, grullas, reds,
yellows, oranges, browns, and blacks seen in Texas Longhorns come from
varying amounts and patterns of expression of these two pigments on different
parts of the body. However, the distribution of these two pigments is
controlled by a large number of different genes, which makes the inheritance
of the two pigments somewhat complex.
The best-studied gene related to color is the gene that controls the relative
degree of eumelanin and phaeomelanin production. This gene (known as the
Extension gene) regulates the levels of an enzyme called tyrosinase.
Low levels of tyrosinase result in phaeomelanin (red pigment) production,
and high levels of tyrosinase result in production of eumelanin (black
pigment). The wild aurochs of Europe (the ancestors of Texas Longhorns,
as well as other breeds of Bos taurus) are thought to have had
a single common allele for this gene, which is referred to as the “wild-type”
allele. The wild-type allele results in variable but typically intermediate
amounts of tyrosinase. This means that both eumelanin and phaeomelanin
are produced, and the ratio and distribution of the two pigments may be
modified by other genes. Longhorns that have two copies (one from each
parent) of the wild-type allele at the Extension gene are typically
some shade of reddish brown at birth, but often grow darker as they grow
older (and may appear black as adults). The relative expression of eumelanin
appears to be related to the sex of the animal, and males (with wild type
alleles at the Extension gene) are more likely to be black as
adults than are females. However, the muzzle ring of these individuals
is usually light tan or brown rather than pure black (this is often called
“mealy-mouthed,” because it looks as if the animals have been
eating corn meal). Other individuals with the wild-type allele may be
dark brown (including Parker brown), medium brown, or a mixture of brown
and black or red and black (including brindling, wine-colored, walnut,
and many of the other unusual colorations of Texas Longhorns).
There are two well-studied alleles (forms of a gene) that differ from
the wild-type allele at the Extension gene in cattle. These alleles
resulted from small mutations in the DNA sequences for this gene that
were selected by early humans during the domestication of cattle (probably
to make breeds more distinguishable). Mutations in DNA can take several
forms, including substitutions (replacing one “letter” in
the DNA sequence with another) and deletions (removing a letter from the
sequence). Substitutions often change the function of a gene, but may
not render it functionless. Deletions, on the other hand, often destroy
the function of a gene. One of the alleles at the Extension gene
differs from the wild-type allele by a single substitution. This simple
change results in a small change in function, which in turn results in
an excess production of black pigment in skin and hair cells. Because
the hairs have an excess of eumelanin, any colored hairs will be black
at birth (other genes may keep some of the hairs from expressing any pigment,
so the calf is often black and white). Even if a calf inherits just one
copy of this allele, almost any hair that is pigmented will be colored
black. Thus, if a bull (or cow) has inherited copies of this black allele
from both its mother and its father, then all of its own offspring will
also express black (no matter what gene is inherited from the other parent).
This is how some breeders can guarantee that their bulls will always produce
calves with black coloration: the bull has been tested and found to be
homozygous for the black allele at the Extension gene (which
simply means that both of its copies of this gene are the dominant black
allele).
The other common allele at the Extension gene in cattle is a
deletion mutation (a single nucleotide has been lost), which results in
a non-functional gene. If an animal has two copies of this allele, then
that animal lacks the ability to produce eumelanin. Phaeomelanin (red
pigment) is still produced, however, so any pigmented hairs have a basic
red coloration. If an animal has only one copy of the red allele and one
of the wild-type allele, then there is still enough tyrosinase produced
for the coloration to appear just like a homozygous wild-type individual
(i.e., some mixture of black and red pigment, but typically darkening
with age, especially in males). Thus, the wild-type allele is said to
be dominant over the red allele, since an animal with both alleles will
show the wild-type coloration. On the other hand, the black allele is
dominant over both the wild-type and red alleles, since even one copy
will result in an over-abundance of eumelanin. Therefore, a cow or bull
that is black at birth may be homozygous black, or heterozygous black
and wild-type, or heterozygous black and red (any of these combinations
will simply look black). A calf with wild-type coloration may be homozygous
wild-type, or heterozygous wild-type and red. Finally, a true red calf
is always homozygous for the red allele.
The “black” allele is abbreviated ED
(the E stands for Extension, and the subscript D stands
for dominant black), the wild-type allele is abbreviated E+ (the
superscript + symbol is used to designate the wild-type allele at any
given gene), and the red allele is abbreviated e (lower case
is used to indicate that this allele is recessive to the other two alleles).
Geneticists usually indicate the dominance order of these three alleles
by writing ED > E+ > e. With this
shorthand, we can indicate a homozygous black bull by writing that its
genotype is ED/ED,
whereas as heterozygous wild-type/red bull would be said to have the genotype
E+/e. Figures 1-3 illustrate examples of Texas Longhorns of each
of these three basic color classes.
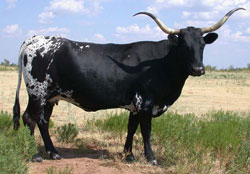
Figure 1. Black coloration
(ED/ED, ED/E+,
or ED/e at Extension)
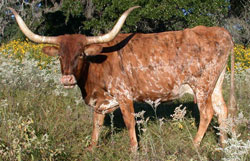
Figure 2. Wild-type coloration
(E+/E+ or E+/e at Extension)
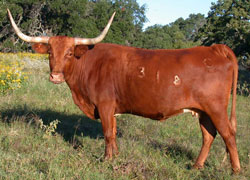
Figure 3. Red coloration
(e/e at Extension)
If these are the three basic colors of cattle, then what
produces colors such as grullas, brindles, duns, light reds or oranges,
and yellows? And how are the different patterns of white coloration produced?
There are eight different genes that are known to affect coloration in
Texas Longhorns, and there are 26,244 different possible genotypic combinations
of known alleles that can appear in an individual bull or cow. In addition,
there are almost certainly many additional genes that affect color and
pattern that have yet to be described, so the number of possible genotypic
combinations for color and pattern is almost certainly many times larger.
Of course, all of these genotypic combinations do not produce distinctly
different color patterns, but this helps to explain why Texas Longhorns
are “more varied than the colors of the rainbow,” as J. Frank
Dobie wrote in The Longhorns. Over the next few months, I’ll
describe what we know about these other genes, and how this information
can be used to predict the inheritance of color in your herd.
Acknowledgements: I thank Dr. Tim Olson of the University of Florida for
helpful discussions of the genetics of cattle coloration. See his articles
listed below on this subject for additional information
Want more detail? Please see the following papers:
Additional
Reading and References
Hillis,
D. M. 2004. The genetics of coloration in Texas Longhorns: Part 2: Grulla,
dun, and other reduced pigment patterns. Texas Longhorn Trails
16(5):76-77.
Hillis,
D. M. 2004. The genetics of coloration in Texas Longhorns: Part 3: The
wild-type color variants. Texas Longhorn Trails, September 2004.
Hillis,
D. M. 2004. The genetics of coloration in Texas Longhorns: Part 4: Spotted,
lineback, color-sided, and White Park patterns. Texas Longhorn Trails,
October 2004.
Hillis,
D. M. 2004. The genetics of coloration in Texas Longhorns: Part 5: Roan
and brockling patterns. Texas Longhorn Trails, November 2004.
Joerg, H.
et al. 1996. Red coat color in Holstein cattle is associated with a deletion
in the MSHR gene. Mammalian Genome 7: 317-318.
Klungland,
H. et al. 1995. The role of melanocyte-stimulating hormone (MSH) receptor
in bovine coat color determination. Mammalian Genome 6: 636-639.
Lauvergne,
J. J. 1966. Génétique de la couleur de pelage dex boivins
domestiques. Bibliographia Genetica 20:1-168.
Olson, T.
A. 1980. Choice of a wild-type standard in color genetics of domestic
cattle. Journal of Heredity 71:442-444.
Olson, T.
A. 1981. The genetic basis for piebald patterns in cattle. Journal
of Heredity 72:113-116.
Olson, T.
A. 1999. Genetics of Colour Variation. In The Genetics of Cattle
(R. Fries and A. Ruvinsky, eds.). Pp. 33-53. CABI Publishing, Wallingford,
United Kingdom.
Robbins, L.
S. et al. 1993. Pigmentation phenotypes of variant extension locus alleles
result from point mutations that alter MSH receptor function. Cell
72:827-834.
|